How are bicycles made? From mine to market
How do you take a lump of iron ore or a tank of petrochemicals and turn it into a bike?
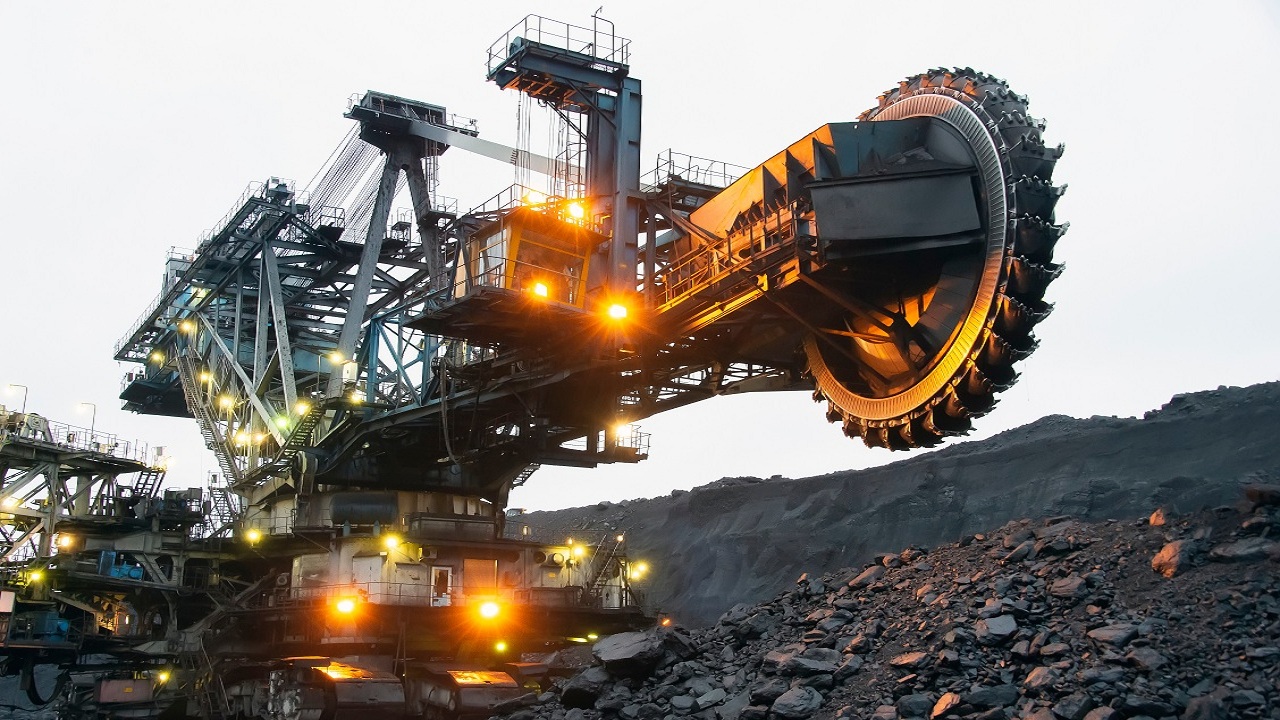
Before I joined Cyclingnews I trained as a Mining Geologist. For the best part of a decade, I worked for companies whose aim was to find new natural resources and dig them out of the ground. One thing that really struck me was, in general, there is an enormous disconnect between the consumer and the production of the goods they consume.
Whether it’s the rare minerals in every smartphone or the platinum in every catalytic converter, the expectation seemed to be that products were produced ‘in a factory’. The same seems to be true for bicycles. You see riders rightly proud of the decal on their seat tube that declares that the bike was ‘Made in Britain’ or ‘Made in U.S.A’, but what does this really mean? Where are bikes ‘made’?
There’s a saying in the mining industry: “If you can’t grow it or catch it you have to dig it up.” It’s a simple phrase, but no less true for it. Each bicycle, whether carbon, steel, aluminium or titanium begins its life as raw materials within the crust of the earth. Before the best road bikes end up on the shop floor they have undergone a great many processes to become something a bike company can turn into a finished product.
Though this will inevitably touch on sustainability, that isn’t the aim of the article, mostly for reasons of concision. Instead I hope to provide an insight into large-scale production.
A note on scale
For many of us bikes make up a pretty big wedge in the pie chart of our lives. They eat up our disposable income and occupy our daydreams. They’re important to us, but in the grand scheme of things the proportion of material that the bicycle industry uses as a proportion of manufacturing as a whole is vanishingly small.
The percentage of iron ore that ends up as high strength, triple-butted tubing is essentially zero when measured against the construction industry. The volume of oil that goes into the production of pre-preg carbon for bicycles is essentially zero compared to that used for fuel or plastics. Aluminium is used for everything from aircraft construction to stovetop coffee pots, and again bicycle production is a small proportion of the whole. Titanium usage is vastly smaller than that of steel, but titanium bicycles are also produced in much smaller quantities than their other metallic siblings.
While what you read below may aid you in making informed purchases, remember that bicycle production as an industry has a much smaller impact than many others, and produces green transportation options and mobility provision for millions. It is, however, one where you can easily vote with your wallet, and every little helps if your aim is a more sustainable future.
The latest race content, interviews, features, reviews and expert buying guides, direct to your inbox!
How are steel bikes made?
We’ll begin with how the best steel road bikes are made, as it is a good primer for the industry as a whole. Steel represents 95 per cent of annual metal use globally, and given that the percentage of carbon in steel is a matter of a couple of per cent at most, we can use iron and steel synonymously when speaking in global terms. The numbers involved can easily make you lose your sense of scale; in 2015 over two billion tonnes of iron ore was extracted from the earth. To put that into context that's the same weight as two million aircraft carriers being dug out of the ground year in, year out.
The biggest producer of iron ore is Australia, followed by Brazil and China. The steel in your custom stainless frame or your mum’s old mountain bike began life as a rusty rock, more than likely in the Australian outback. It was dug out of the ground in some of the largest mines in the world and placed into a train that’s 1.5 miles long for a journey across the desert to a smelter on the coast.
Mining, as you might imagine, is relatively energy intensive. The mining industry consumes roughly 10 per cent of global energy, and given the energy use within the sector it means four per cent of all the energy produced globally goes into crushing rocks, and once you get it out of the ground you have to turn it from an ore into a metal. This process, known as smelting in the case of most metals including steel, takes the ore or metallic concentrate (a powder that’s relatively high in metal content, more so than the rock it came from), and heats it up to the point the chemical bonds break down, separating iron from the waste, known as slag. The process has basically remained unchanged since the iron age, but has been significantly scaled up. Adding carbon at this point produces steel, and other metals such as molybdenum are added to create specific alloys. To smelt enough steel for a single 1.5kg bike frame requires the same amount of energy as a standard UK household uses in a day.
Consumers nowadays like the ability to trace their consumables back to the source, but in the case of steel, and other metals, this isn’t really possible. Smelters intake ore from mines both domestically and overseas depending on their location and global production, and once it’s gone in the melting pot together there isn’t any way to know what has come from where; It’s like making a cake from four international eggs and trying to work out which country the egg in your slice came from.
We’ve got steel now, right? Then let's make some bikes! Alas, not quite. The raw materials have already potentially travelled hundreds or thousands of miles to the smelter to be turned into steel, but smelters, if they produce any products at all, tend to only make the big stuff - think girders and railway tracks. Otherwise they sell their raw steel to secondary producers, which will likely involve further transport to a factory where, for the purposes of bicycle manufacture, seamless steel tubing is produced.
These seamless tubes of specific high-strength alloy are then shipped to a tubing manufacturer, such as Reynolds in Birmingham, UK, where we can begin to see things becoming bicycle-shaped. I was lucky enough to have a chat with Reynolds to go over some key points.
I imagine for some of you, the above information might have been eye opening about the global resources required to make some metal, but speaking to Reynolds gave me some cause to be optimistic. The main takeaway from our chat was that, considering the iron age began in 1200BC, the knowledge of re-alloying old metals is extremely well established. As such, all of Reynolds steel tubing at 853 level and above (those with raw tubing imported from Germany) is 100 per cent recycled material. Those below 853 are produced in Taiwan and China, as is likely true for much of the industry as a whole, and is majority recycled. Exact figures are hard to quantify, primarily due to China's reticence to share its data.
That’s not to negate the last number of paragraphs; the virgin steel must be produced from raw materials at some point, but the fact it can be recycled near-infinitely, and that it is, are both significant plus points when viewing steel as a material for bicycle construction.
The straight-gauge tubes arrive at Reynolds and are butted to their chosen profiles before finally being sent to a frame manufacturer who will then mitre and weld or braze them into a finished product. If you discount the energy cost of producing the raw steel, given its recycled status (one for debate), the majority of the energy cost comes from transport.
For the most energy-efficient steel, you’d look to an 853 tubeset - raw materials sourced from Germany - built into a bike by a UK manufacturer and sold in a UK showroom. At the other end of the spectrum you could easily imagine a scenario where raw materials from the Far East were finished into tubing in Birmingham, shipped to Taiwan to be made into a frame, before being shipped back to a European showroom.
How are aluminium bikes made?
How does that compare to what's needed to produce the best aluminium road bikes?
Aluminium occupies a middle ground in industrial terms between the simplicity but gargantuan scale of steel, and the extreme complexity of titanium production. It is the most common metallic element found in the crust of our planet, but this doesn't mean you can just pick up a shovel and start digging; in order to produce pure aluminium you need deposits known as bauxites. These are, in simple terms, rocks and soils rich in aluminium oxide. They form in tropical and sub-tropical environments, where the additional heat and moisture chemically weathers away the weaker waste minerals and effectively concentrates aluminium in the environment naturally.
An added bonus of this process is that the deposits occur at or very near the surface, making mining them a (relatively) easy task. China, followed closely by Australia, leads the way in global bauxite production.
Once the bauxite is out of the ground it needs to be converted into alumina before it can be finally refined into usable ingots. This is done using the Bayer process, by dissolving the bauxite in caustic soda at a high temperature and then allowing it to cool. The alumina (aluminium oxide) wants to stay in solution more than the waste products, and so all the waste settles to the bottom as a red sludge, allowing the alumina to be separated off, and then heated to remove any remaining water in the chemical structure.
Now the alumina can be turned into pure aluminium by the process of electrolysis, rather than smelting. The alumina is dissolved in molten cryolite (a solvent mixture of hydrofluoric acid, aluminium hydroxide and bicarbonate of soda) in enormous graphite-lined tanks. Graphite rods are suspended in these tanks and a constant current 1,000 times greater than that required to start a car is passed through the liquid. At the negatively charged base of the tank aluminium atoms dissolved in the cryolite receive electrons to produce pure liquid aluminium, which can be drawn off intermittently using a giant vacuum. Conversely at the positive rods, oxygen ions lose electrons and react with the graphite to produce carbon dioxide.
If you’re sitting there thinking “I bet pumping 400,000 amps through a tank of 1,000 degree liquid sure uses a lot of energy!” you’d be right; to produce aluminium from a typical mix of 80 per cent virgin material and 20 per cent recycled material requires 22 times the energy consumption as that of recycled steel. For this reason, electrolysis plants are usually located right next to a power station. In countries with renewable energy this could be next to a hydroelectric plant. In the case of China, 93 per cent of aluminium production derives its energy from coal fired power stations.
Aluminium is easily recycled, and has a well established industry around the process. Producing purely recycled aluminium is, to all intents and purposes, the same energy consumption as that of recycled steel. Unless publicised, it is likely that the aluminium in any given bicycle tube is derived from a mix of virgin and recycled material.
In the same way as steel and titanium, the raw aluminium is manufactured into tubing from raw materials and subject to the same levels of global transport, before further shipping to bicycle manufacturers. Aluminium is usually shaped by a process of hydroforming, where ultra-high pressure water is forced into the inside of the tube while it is housed in a mould, allowing deviation from the traditional round cross sections of steel and titanium.
How are titanium bikes made?
Despite what many riders would regard as the most exotic of the mainstream bicycle materials, titanium is the fourth most abundant structural metal element in the earth’s crust (behind iron, magnesium, and aluminium). Why, then, is titanium tubing so much more expensive than the equivalent in steel or aluminium?
While for steel you essentially melt a rusty rock and throw in some coal, and for aluminium you throw aluminium oxide into a electrified bath, getting a usable lump of titanium is significantly more involved.
Extraction of the raw material involves separating grains of sand, mercifully in an automated process. The main ore mineral, an iron-titanium oxide, exists in ancient and current river deposits - basically dried up old river beds. These old river sands are, whimsically, poured down a giant helter-skelter slide, and as the titanium-rich grains are heavier than grains of quartz they eventually separate into a single stream that can be siphoned off for extensive further processing.
The iron in the iron-titanium oxide is, chemically speaking, in the way, and is first removed either by heating to 1,600 degrees Celsius with a sprinkle of high grade coal, or dissolved away by boiling hot hydrochloric acid to leave titanium oxide. 95 per cent of this TiO2 is used as a white pigment, but the five per cent destined to become a shiny silver lump must undergo a few more steps.
The remaining five per cent undergoes what is industrially known as the Kroll Process. The Titanium ore is heated in a furnace in the presence of chlorine gas to produce gaseous titanium tetrachloride, which is then cooled and condensed into a liquid, known colloquially as Tickle due to the chemical formula, TiCl4.
Given you can’t make a bike out of liquid, this Tickle is then heated for four days in the presence of magnesium, which causes the chlorine to switch allegiances and bond to the more reactive magnesium, leaving solid titanium. The glowing hot tank of chemicals then has all of the air sucked out of it to create a vacuum, drawing off the magnesium chloride and creating a titanium sponge full of holes.
This sponge is then crushed to a coarse powder before being compacted into a near-solid block by a giant hydraulic press. It can, finally, then be melted down into an ingot and manufactured into things, mostly by the aerospace industry.
As you might imagine, this process is relatively energy intensive. To get titanium metal from a concentrated ore requires 60 times as much energy as to produce the same weight of steel from recycled steel. Though this isn't necessarily comparing like with like, steel has a well formed recycling network on a global scale, which titanium lacks.
As with steel, the majority of the metal produced doesn’t end up in high-end bicycles. The majority goes into things like aeroplanes, with an Airbus A380 requiring 77 tonnes of the stuff. Though steel is easily recycled, given the increased cost of production, the industry is far less wasteful with titanium. A great many aerospace parts are milled from solid ingots, and the swarf (chips of metal) is all collected and re-cast into metal. Speaking again to Reynolds, who also produces titanium tubesets, it transpires that 100 per cent of its titanium tubing is made from aerospace waste.
If you’re interested in the source of your titanium, it will likely have started life in China, who produces more than double the next biggest national producer, South Africa. Naturally, as with aluminium and steel, the raw materials will also have spent significant time in transit across the globe.
How are carbon fibre bikes made?
As a bit of light relief from the big numbers involved in the production of iron and steel we can pivot to the gargantuan world of hydrocarbon extraction. Carbon fibre, both the carbon fibres themselves and the resins within which they are set, are products of the petrochemical industry, and as such rely on the extraction of oil. Oil is, after all, just various carbon chains in a thick soup.
The extraction of oil is slightly more familiar to the average person than the intricacies of the mining industry. An oil rig, whether on land or sea, drills into an underground reservoir of the black stuff and pumps it out. Naturally it’s more involved than that, but without going off on unnecessary tangents that’s all that’s really necessary to know from a material extraction point of view.
The oil industry is, to a great extent, responsible for the materials on which we rely for daily life, from the plastics in our water bottles to the helium in our birthday balloons. Making a carbon fibre frame is very chemistry heavy, and so I’ll try and generalise as much as possible.
The best carbon road bikes, carbon-fibre frames and components, are more or less composed of two parts: the fibres themselves, and the resin in which they are set. Both are derived from oil. Carbon fibres start life as a vat of chemicals (solvents, catalysis, and the building blocks of a polymer chain - monomers). In much the same way as nylon threads are produced, polymer threads are spun out of this monomer-rich liquid, and stretched to the desired thickness, and then rinsed of any residue.
At this point they’re what can be thought of in general terms as similar to nylon. Strong, flexible, but not much use for making a bicycle. To convert the plastic polymer threads into carbon they are roasted at a high temperature, either in an unreactive atmosphere or under a vacuum, at which point the polymer atoms ‘carbonise’. Now you’ve got a long thread of carbon, which can either be woven into a mat, or laid parallel to other fibres in what is known as unidirectional carbon fibre.
The resins in which the fibres are set are also derivatives of the oil industry, but the chemical processes involved in producing epoxy are a little more complex, and a lot less exciting. Just know that the entirety of a high end carbon frameset, the carbon wheels, and any other finishing kit began life deep underground as a decomposing ancient swamp.
The sheets of woven carbon fibres are often impregnated with heat-curing resin, into what is known as pre-preg carbon. These are placed into a mould in specific orientations so as to produce strength or flexibility where the manufacturer would like it. Once a whole bicycle has been laid up, the mould is baked, and inside a rubber bladder is inflated so as to press the fibres to the edges. Once the baking has cured the resins, a complete frame, or another component can be removed for finishing.
The oil industry, quite rightly at times, has a bad reputation in some circles. The percentage of oil that actually goes into making bike frames, however, is so vanishingly small that choosing to avoid a carbon frame as a two finger salute to Big Oil is sadly a slightly futile gesture. Enough oil is produced globally to fill four Olympic swimming pools every minute, 24 hours a day, 365 days a year. Assuming five kilograms of carbon fibre in a complete race bike, the oil industry could churn out 33 complete bikes every single second.
You hear people refer colloquially to carbon bikes as ‘plastic’ as a joke, but it’s a joke that’s perhaps a little too close to the bone if you’re a fan of reducing your waste. Steel, aluminium, and, with a little more difficulty, titanium frames can be recycled into new bikes, girders, drinks cans or aeroplanes. Carbon fibre though, like so many plastics, is remarkably resistant to breaking down. Though the energy needed to produce a carbon frame is lower than that of metal alternatives, there is no way to recycle a carbon frame, and as such at the end of its lifespan it is destined to finish out its days in landfill, where it will remain for tens of thousands of years.
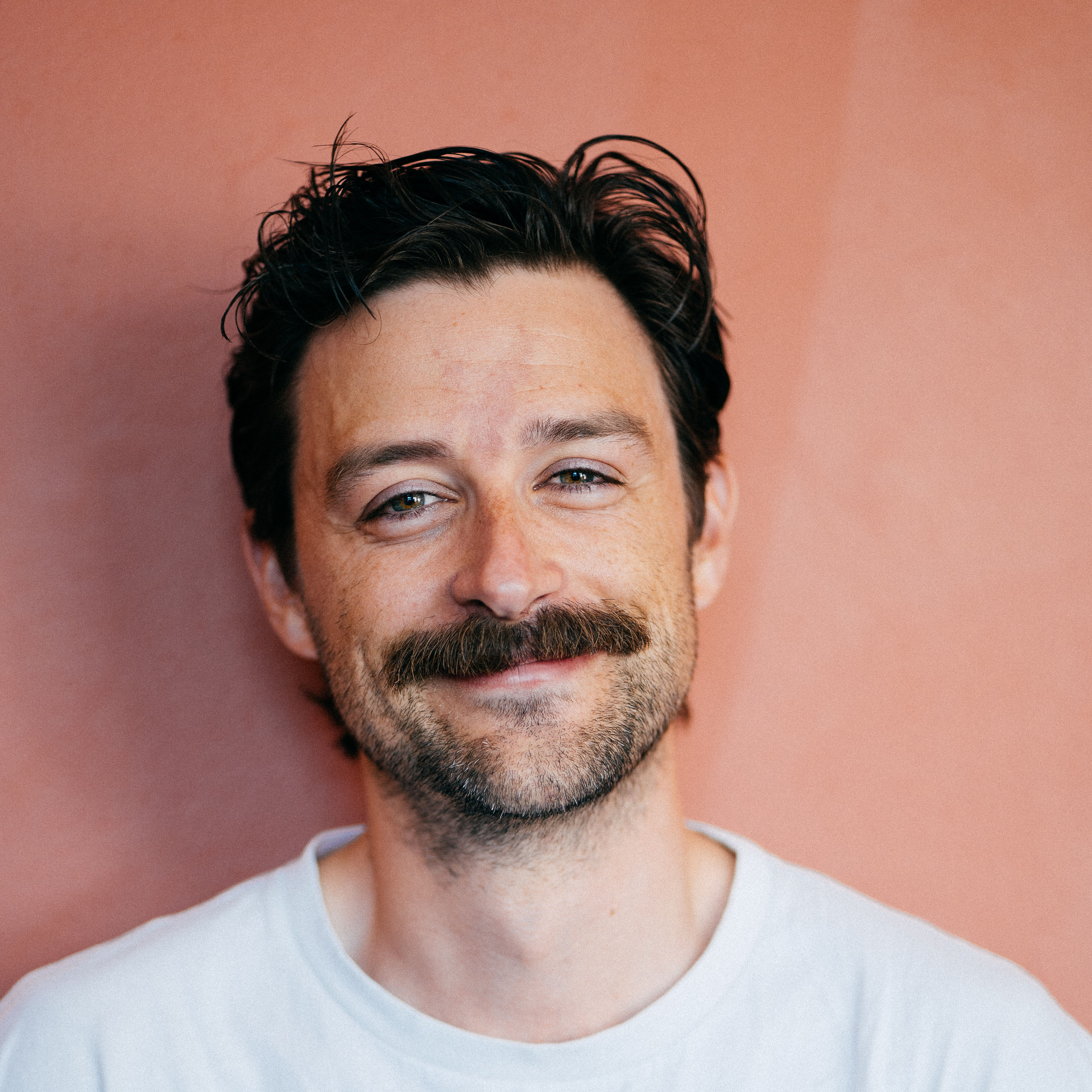
Will joined the Cyclingnews team as a reviews writer in 2022, having previously written for Cyclist, BikeRadar and Advntr. He’s tried his hand at most cycling disciplines, from the standard mix of road, gravel, and mountain bike, to the more unusual like bike polo and tracklocross. He’s made his own bike frames, covered tech news from the biggest races on the planet, and published countless premium galleries thanks to his excellent photographic eye. Also, given he doesn’t ever ride indoors he’s become a real expert on foul-weather riding gear. His collection of bikes is a real smorgasbord, with everything from vintage-style steel tourers through to superlight flat bar hill climb machines.